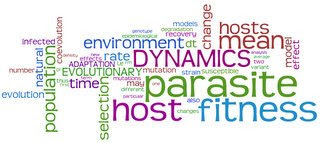
We recently read a theory paper by Gandon and Day (2009). In this paper they describe a valuable method for dissecting how interactions between a host and parasite alter mean fitness. Their method uses an understanding built from Fisher's fundamental theorem. They partition changes in mean fitness based on three different factors: natural selection, environmental change, and mutation. We know that the rate of adaptation is going to result from the amount of genetic variance in the focal organism (Fisher's theorem), but what about the impact of an interacting species that evolves as well (i.e. a coevolving parasite? Here is the real beauty of their analysis because the coevolving species becomes the environment. By separating the changes in a population mean fitness into changes driven by different forces, the authors provide not only a mathematically useful model, but also a useful intuition for understanding how hosts and parasites coevolve.
There are several ways that theoreticians often describe a host-parasite interaction (e.g. gene-for-gene, matching alleles) and these describe natural systems to some degree of accuracy. The authors use their method to analyze some recent empirical evidence (Buckling and Rainey 2002; Decaestecker et al 2007). They use the time series data on the interaction to test hypotheses of the nature of the interaction. These empirical studies compare the fitness of parasites against hosts from the past that they have coevolved with and those from the future (hosts that evolve later in the study). By making these comparisons, they have the ability to hold other factors constant (the genetic variance of the parasite population) and vary the environment (the hosts). Their model makes different predictions that should be evident from empirical evidence about how parasite mean fitness should change when the environment is varied.
The authors very elegant proposed method of looking at changes over time works well for systems where archives of past populations are possible as in experimental evolution systems (Buckling and Rainey 2002) or clever natural systems (Decaestecker et al 2007), but what about the rest of us? Addressed in at the very end, but only briefly, is a comparison of spatial patterns of coevolution when temporal data is missing. I think this issue of substituting space for time is potentially very powerful, but also somewhat more complicated. Temporal samples of a coevolutionary system could be predicted to have a certain amount of autocorrelation, but does this hold for spatially distributed systems. It certainly would nice to assume that there is a relationship between distance and time and this will of course depend on gene flow. How would selection mosaics (Gomulkiewicz et al 2007; Thompson 1999, 2005) impact this potential relationship? I look forward to future research as it provides some answers.
References
Buckling, A., and P. B. Rainey. 2002. Antagonistic coevolution between a bacterium and a bacteriophage. P Roy Soc Lond B Bio 269:931-936.
Decaestecker, E., S. Gaba, J. A. M. Raeymaekers, R. Stoks, L. Van Kerckhoven, D. Ebert, and L. De Meester. 2007. Host-parasite 'Red Queen' dynamics archived in pond sediment. Nature 450:870-873.
Gandon, S., and T. Day. 2009. Evolutionary epidemiology and the dynamics of adaptation. Evolution 63:826-838.
Gomulkiewicz, R., D. M. Drown, M. F. Dybdahl, W. Godsoe, S. L. Nuismer, K. M. Pepin, B. J. Ridenhour, C. I. Smith, and J. B. Yoder. 2007. Dos and don'ts of testing the geographic mosaic theory of coevolution. Heredity 98:249-258.
Thompson, J. N. 1999. Specific hypotheses on the geographic mosaic of coevolution. American Naturalist 153:S1-S14.
Thompson, J. N. 2005.
The Geographic Mosaic of Coevolution. University of Chicago Press, Chicago.
Paper read
Gandon, S., & Day, T. (2009). EVOLUTIONARY EPIDEMIOLOGY AND THE DYNAMICS OF ADAPTATION Evolution, 63 (4), 826-838 DOI: 10.1111/j.1558-5646.2009.00609.x
No comments:
Post a Comment